Of Microbes and Mangroves: PNS Bacteria and Rhizophora mangle
- Taras Pleskun
- Dec 17, 2023
- 11 min read
Updated: Nov 11, 2024
Mangroves are one of those keystone species which have the potential to redefine the greater aquarium hobby. Mangroves are organisms which exist in all three realms: soil, water and air. Within each of these realms, their growth establishes structure and stability which in turn fosters a myriad of other organisms. The stems and branches of mangroves house the nests of birds and act as an arboreal highway for reptiles, insects and mammals. The roots of mangroves form a network of bioactive pillars in the water column. Each submerged mangrove root becomes a glorious biometropolis of attached bivalves, macroalgae, barnacles, tunicates, sponges and anemones. Every inch of these sunken skyscrapers writhes with darting copepods, skittering crabs and planktivorous fish. These roots penetrate both the shallowest and deepest parts of the sediment. There they bring with them a world of pioneering microbes eager to colonize the darkest parts of earth. The microbes that follow mangrove roots into those depths thrive where there is no light, no oxygen and all manner of toxic things. Still, the byproducts of their growth nourish the mangrove, bringing benefit to all other life above.
The red mangrove (Rhizophora mangle) is of particular interest to reef aquarists as it is the estuarine mangrove: The bridge between saltwater and freshwater. The inevitable increase of enthusiasm for mangroves will likely manifest into awesome things such as outdoor mangrove/reef ponds, specialized mangrove-based refugia and estuarine paludaria…there may even be a day when mangrove sculpture gardens and formal aquascapes exist. The only barrier from these glorious realities is an increased, intimate understanding of mangrove biology and the microbiome which supports it. When this understanding is deployed through practical husbandry techniques, mangroves will provide the saltwater aquarium hobby with a forest of possibility.
Purple non-sulfur bacteria (PNSB) are one of the most prolific microbes found in wild mangrove ecosystems worldwide. This makes reasonable sense as the PNS bacteria as a whole are known for their adaptability and metabolic plasticity. For example, Rhodopseudomonas palustris can survive in freshwater, marine and terrestrial ecosystems. It possesses a ‘swiss-army knife’ metabolism, allowing it to respire with or without oxygen, and derive energy from various organic and inorganic carbon, nitrogen, phosphorus and sulfur compounds. Not only are various PNSB species found in mangrove soils, but they have been observed occupying the rhizosphere of red mangroves.

The rhizosphere refers to a specialized zone of plant root tissue essentially designed to encourage the growth of beneficial microbes. The rhizosphere is a plane of commerce where localized resource exchange can occur between microbe and mangrove cells. This collective exchange is absolutely essential for the mangrove to efficiently acquire resources from the soil. Much like a mobile factory, the mangrove’s prop root acts to transport microbial workers to areas of abundant raw material. In return for housing, transportation and sugars, the mangrove host is provided with the fruits of the microbe’s metabolic labor. In some circumstances, the microbes directly provide a favorable product such as carbon, iron, vitamins, phytohormones etc. The activity of microbes can also eliminate toxins, creating a more favorable environment for other mangrove symbiotes. Other microbes ward off pathogens that may attack mangrove cells. By such collective benefit, symbiotic microbes (such as PNS bacteria) are integral to the successful growth of Rhizophora mangle.
Successfully growing mangroves in captivity is an endeavor that fuses horticulture and aquaculture, sentiments of the gardener and of the aquarist. And as every gardener knows–it’s all in the soil. Naturally, light and water quality dictate a mangrove’s growth, but it is the soil which sways water quality and it is the nutrients acquired from the sediment which allows the mangrove to utilize light of any kind. Therefore…
The battle is won and lost in the soil.

The mangrove is an organism which creates many worlds for many other critters. Likewise, the nutrition of a mangrove depends on the success of millions of microworlds. Much like a coral, a mangrove is nothing without a supporting entourage of beneficial bacteria, algae, fungi and archaea. Keeping the symbiotes happy is key to keeping the ‘big tree’ healthy. The successful mangrove aquarist/gardener must acknowledge and appreciate the diverse microbiome which participates in the flourishing of a robust Rhizophora mangle specimen.
Activities conducted within the rhizosphere and surrounding soil particles include but certainly are not limited to the following.
Heterotrophy
Heterotrophy is the process by which microbes consume organic carbon. Wild mangrove
estuaries are incredibly dense with organic carbon from marine sediments, terrestrial runoff and decomposing organisms. This organic carbon must be consumed by soil microbes so that carbon dioxide can be amply available to mangrove cells. Plant cells use carbon dioxide for photosynthesis and release oxygen as a byproduct. Aerobic heterotrophic bacteria are limited by how much oxygen is available. Mangroves may be able to deliver localized oxygen to microbial heterotroph populations within the rhizosphere, allowing them to operate even well below the aerobic layers of the sediment. Heterotrophy also directly affects the C:N balance in the sediment…having further cascading consequences in both mangrove and microbe metabolism.
Diazotrophy (i.e., Nitrogen Fixation)
Nitrogen is a primary limiting nutrient for photosynthetic growth. This can be especially true
when wild mangroves are transferred into a captive environment. No display aquarium will
likely have a shade of the nitrogen resources available to a wild mangrove. Feller et al 1995
demonstrated how nitrogen limitation can induce dwarfism in Rhizophora mangle. Although this offers the prospect of mangrove bonsai, which would be of a more manageable size for home aquaria, it also addresses a likely deficiency that must be accounted for.
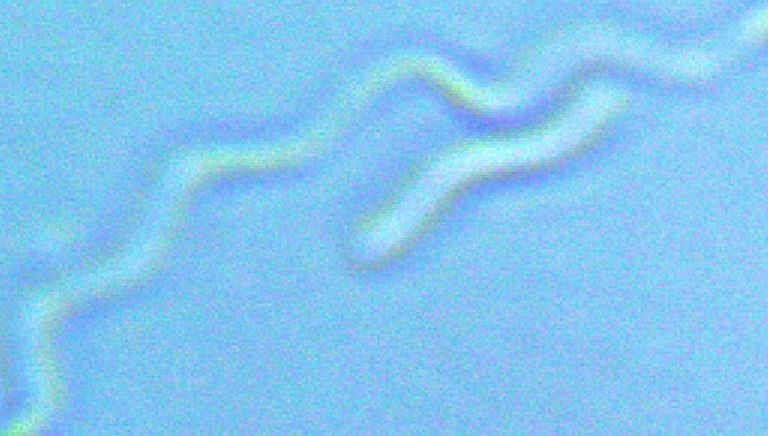
Diazotrophs are microbes which can fix atmospheric nitrogen (N2) into ammonia (NH3), which can then be utilized to build tissues (particularly amino acids, which are the building blocks of proteins). Diazotrophs act as ‘fuel-injectors,’ providing nitrogen directly to their mangrove host, which is largely unavailable in the outside environment. The observation of such activity on wild mangroves suggests that there may be effective pathways to get consistent mangrove growth even in lower-nutrient display systems. The fact that diazotrophs require anaerobic zones to conduct their chemistry suggests specialized zones exist within the mangrove rhizosphere to house and facilitate this set of microbial symbionts.That notwithstanding, PNS bacteria such as Rhodopseudomonas (Family Bradyrhizobiaceae) generally can associate with any plant species, including legumes, whereas certain, more famous, relatives that are obligate symbiotes (Family Rhizobiaceae) are often restricted to the specialized root nodules of legumes.
Phosphate Solubilization
Phosphorus is another common limiting factor for conducting photosynthesis. Phosphorus itself is abundantly available within sediment, but in an insoluble form which plant cells cannot utilize. Certain bacteria, such as Rhodopseudomonas palustris, are capable of ‘acid-mining’ these phosphorus sources receiving energy from the conversion of insoluble phosphorus. The byproduct is soluble phosphate which can be readily utilized by the mangrove cell. Indeed, Rhodopseudomonas palustris is already commonly used in both indoor and outdoor agriculture (ranging from PGA golf courses to the cannabis industry) for this purpose.
Vitamin Synthesis
Cobalamin, or Vitamin B12, is an essential vitamin for eukaryotic cells. Oftentimes, it must be
provided directly by bacteria or other microbial intermediates. Furthermore, various cells utilize slightly different forms of cobalamin better than others. Rhodopseudomonas palustris has been observed producing high levels of cobalamin. Excess cobalamin production produced by PNS bacteria can have profound growth-enhancing effects on both the mangrove host cell and surrounding beneficial symbionts.
Sulfur Oxidation
Sulfides are a dominant sulfur product in mangrove sediments. Hydrogen sulfide is toxic to
many cells in excess, and without an active force mitigating it, would make much of the life in
wild mangrove forests impossible. Certain bacteria, such as Rhodopseudomonas palustris, are capable of oxidizing moderate concentrations sulfides into less toxic sulfur ions and sulfate compounds (it is the distantly related purple sulfur bacteria (PSB) that are more adept at that task, as their name suggests). In addition to mitigating the spread of toxic sulfides, this collective conversion is a major producer of energy for these microbes and their associated symbionts.
Siderophore Synthesis
Iron is another important limiting element for plant growth. Many photosynthetic systems
quickly become iron deficient unless they can find a way to localize a source of iron.
Furthermore, each cell has specific forms of iron they are best designed to utilize. Some microbes, such PNSB, produce siderophores as a means of collecting iron outside the cell. These increasing concentrations of localized iron become accessible not only for the microbes, but also the mangrove cell which houses them. Siderophores may also be responsible for the acquisition of zinc and other trace metals needed for mangrove metabolism.
Phytohormone Synthesis
Some microbes have been documented directly secreting growth-stimulating hormones to their mangrove host. Batool et al 2017 observed Rhodopseudomonas spp. bacteria providing auxin directly to the roots of Rhizophora mangle. Such complicated and nuanced, phytohormonal exchanges warrant their own article, but needless to say, such exchanges surely play a decisive role in the timing of mangrove growth as well as delineation of resources between host and its various symbionts. The strategic secretion of phytohormones may also be a means of PNSB, and other symbionts, to ‘guide’ the direction of root growth towards areas of abundant resources. It may also be a means by which microbes manipulate the growth architecture of the rhizosphere within the mangrove root so as to better suit the needs of their populations.
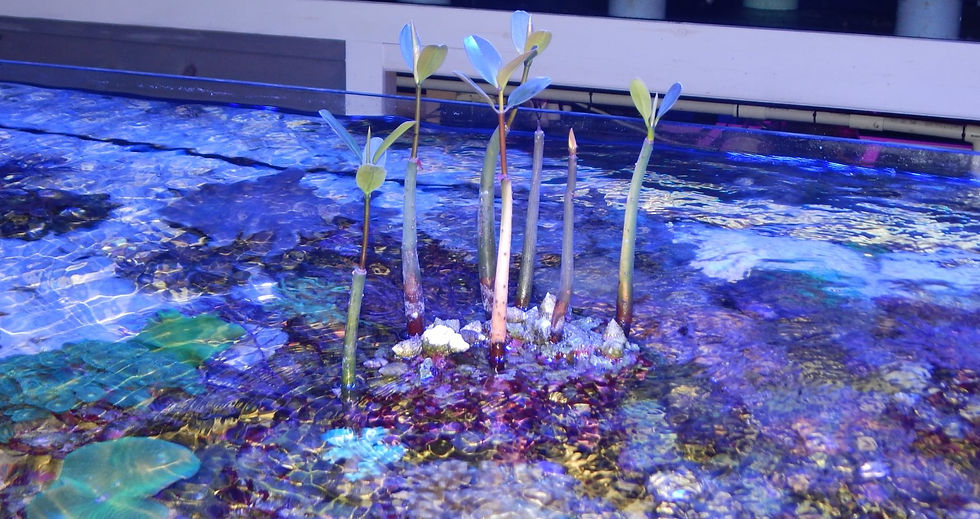
Potential to Inhibit Mangrove Pathogens
Beneficial microbes are on the frontlines of preventing disease in a mangrove cell. Due to their intimate relation and entangled bioeconomic dependence, it is overwhelmingly within the best interest of symbiotic microbes to discourage, control and eliminate potential pathogens. Competition for resources, formation of shield-like biofilms and secretion of antibiotics are all means by which Rhodopseudomonas palustris has been demonstrated to combat various forms of Vibrio bacteria. Furthermore, it should be noted that bacterial pathogens such as Vibrio require mass signaling and communication in order to manifest disease. Beneficial microbes have the ability to jam these signals (quorum inhibition) in a way that controls potential troublesome bacterial populations.
Facilitation of Greater Mangrove Community (Nitrification, Denitrification, Photosynthesis etc.)
The mangrove ecosystem is one which is not composed solely of microbes and plant cells.
Every other organism which regularly inhabits the mangrove forest contributes in some way to the collective stability of that system. The growth of oysters, barnacles and other sessile filter-feeders slow the flow of water around mangrove stands, allowing for more sediment to
collect at their roots. Copepods, amphipod and other grazers remove excess organic matter and attract fish, which contribute a valuable source of bioactive nitrogen. All of these creatures contribute to the flow of resources in and out of the mangrove’s sphere of biological influence. Therefore, it should not be ignored that various metabolic processes occur within the mangrove rhizosphere which facilitate the existence of these larger inhabitants.
PNS bacteria are known for their ability to photosynthesize in anaerobic zones. This consumption of CO2 also aids in pH stabilization. PNS bacteria utilize carotenoids and other pigments to photosynthesis. These pigments are of profound nutritional value to any organisms which consume them and they perpetuate (in the form of intense coloration) up the food chain. PNSB cells also conduct denitrification, allowing them to eliminate rising contractions of nitrate, which could ail various marine animals and promote the growth of undesirable algae. In addition to the elimination of hydrogen sulfide, Batool et al 2017 observed the ability of Rhodopseudomonas spp. to conduct redox reactions to detoxify arsenic.
Practical Application of PNS
It is by such metabolic criteria that certain naturally occurring mangrove symbionts (such as PNS bacteria) have been isolated and cultivated with the intention of harnessing the power of wild mangrove soils! PNS bacteria, such as Rhodopseudomonas palustris, are capable of conducting all of these metabolic reactions should environmental conditions allow. Furthermore, they will ‘toggle’ in between metabolic activity depending on where they are present in the rhizosphere, and what resources are in relative abundance. This plasticity is what makes Rhodopseudomonas an ideal probiotic candidate for mangrove propagation and growth. The question…and fun begins…when one considers which practical husbandry practices best cater to the mangrove microbiome desired; microbial stability leads to mangrove stability and this leads to the success of many many larger animals…all collectively adding to the growth, stability and majesty of the mangrove forest.
Every controllable factor in the captive setting (water level/quality, light spectrum/intensity, soil composition etc.) all have dramatic effects on the mangrove’s choice in rhizosphere composition and therefore the composition of microbes it will house and the metabolic activities they will conduct. For example, Ellison et al 1997 observed how low-water mangroves demonstrated significantly higher root porosity–a feature associated with beneficial microbes. Another study, conducted by Batool et al 2017, experimented with the inoculation of mangroves with different PNS bacteria and compared their growth activity. This study reported a consistent increase in growth (both shoot and root length) amongst red mangroves inoculated with PNSB (Rhodopseudomonas palustris and Rhodopseudomonas faecalis) compared to the uninoculated control. Therefore, there may be extreme benefit to pre-inoculating mangrove propagules/saplings in a PNSB broth before placing it in the substrate of an aquarium. Larger scale ponds and paludariums generally may benefit from periodic dosing of concentrated PNSB cultures, allowing the probiotic to colonize the soil and water column.
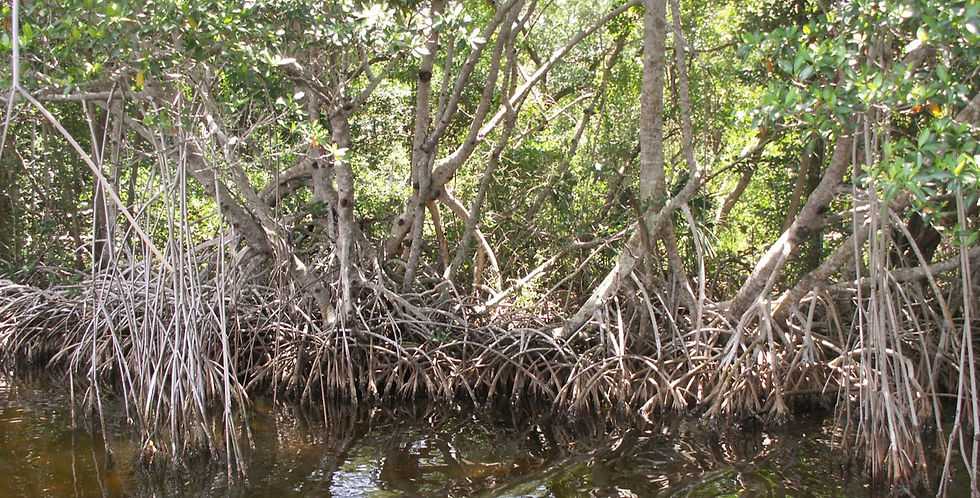
To successfully rear a mangrove propagule into a charismatic tree requires the creation of three suitable environments amongst the earth, water and air. Victory in this pursuit has rewards far beyond a growing mangrove plant. For in the process, three general habitats have been created…granting the aquarist triple the canvases in which to house various other plants, fish, reptiles, amphibians and invertebrates. Ever more complex biological architecture opens a new world of aquascaping possibilities. The major obstacle to this wonder, is the ability to successfully establish ‘magic mangrove mud’ de novo in a captive environment. Whereas, wild mangrove propagules depend on recruiting microbes during this time afloat, captive propagules will likely greatly benefit from strategic early dips with proven mangrove probiotic bacteria species. Due to their metabolic plasticity and vast array of beneficial abilities, the purple non-sulfur bacteria will adapt and grow with the mangrove on its long journey from propagule to tree. Such intimate and powerful relationships are the ones from which beautiful new worlds arise.
Literature Consulted
Azira, Z., Hafizah, N., Rahman, M. M., Kamaruzzaman, B. Y., & Faizul, N. (2014). Carotenoid Contents in Anoxygenic Phototrophic Purple Bacteria, Marichromatium Sp. and
Rhodopseudomonas Sp. of Tropical Environment, Malaysia. Oriental Journal of Chemistry,
30(2).
Bashan, Y., & Holguin, G. (2002). Plant growth-promoting bacteria: a potential tool for arid mangrove reforestation. Trees, 16(2-3), 159-166.
Batool, K., & Rehman, Y. (2017). Arsenic-redox transformation and plant growth promotion by purple nonsulfur bacteria Rhodopseudomonas palustris CS2 and Rhodopseudomonas faecalis SS5. BioMed research international, 2017. Behera, B. C., Mishra, R. R., Dutta, S. K., & Thatoi, H. N. (2014). Sulphur oxidising bacteria in mangrove ecosystem: A. Cabral, L., de Sousa, S. T. P., Júnior, G. V. L., Hawley, E., Andreote, F. D., Hess, M., & de Oliveira, V. M. (2018). Microbial functional responses to long-term anthropogenic impact in mangrove soils. Ecotoxicology and Environmental Safety, 160, 231-239. Ellison, A. M., & Farnsworth, E. J. (1997). Simulated sea level change alters anatomy, physiology, growth, and reproduction of red mangrove (Rhizophora mangle L.). Oecologia, 112(4), 435-446.
Ellison, A. M., Farnsworth, E. J., & Twilley, R. R. (1996). Facultative mutualism between red mangroves and root‐fouling sponges in Belizean mangal. Ecology, 77(8), 2431-2444.
Feller, I. C. (1995). Effects of nutrient enrichment on growth and herbivory of dwarf red mangrove (Rhizophora mangle). Ecological monographs, 477-505.
Gill, A. M., & Tomlinson, P. B. (1969). Studies on the growth of red mangrove (Rhizophora mangle L.) I. Habit and general morphology. Biotropica, 1-9.
Gill, A. M., & Tomlinson, P. B. (1971). Studies on the growth of red mangrove (Rhizophora mangle L.) 3. Phenology of the shoot. Biotropica, 109-124.
Gill, A. M., & Tomlinson, P. B. (1977). Studies on the growth of red mangrove (Rhizophora mangle L.) 4. The adult root system. Biotropica, 145-155.
Gotto, J. W., & Taylor, B. F. (1976). N2 fixation associated with decaying leaves of the red
mangrove (Rhizophora mangle). Applied and Environmental Microbiology, 31(5), 781-783.
Gros, O., Bisqué, L., Sadjan, M., Azede, C., Jean-Louis, P., & Guidi-Rontani, C. (2018). First description of a new uncultured purple sulfur bacterium colonizing marine mangrove sediment in the Caribbean: Halochromatium-like PSB from Guadeloupe. Comptes Rendus Biologies, 341(7-8), 387-397.
Hansen, T. A., & van Gemerden, H. (1972). Sulfide utilization by purple nonsulfur bacteria. Archiv für Mikrobiologie, 86, 49-56.
Nupur, P., Srinivas, T. N. R., Takaichi, S., & Anil Kumar, P. (2014). Rhodovulum mangrovi sp. nov., a phototrophic alphaproteobacterium isolated from a mangrove forest sediment sample. International journal of systematic and evolutionary microbiology, 64(Pt_9), 3168-3173.
Rao, C. R., & Krishnamurthy, K. (1994). Chemoheterotrophy in the mangrove environment. Current Science, 382-385.
Sahoo, K., & Dhal, N. K. (2009). Potential microbial diversity in mangrove ecosystems: a
review. de Sousa, S. T. P., Cabral, L., Lacerda-Júnior, G. V., Noronha, M. F., Ottoni, J. R., Sartoratto, A., & de Oliveira, V. M. (2020). Exploring the genetic potential of a fosmid metagenomic library from an oil-impacted mangrove sediment for metabolism of aromatic compounds. Ecotoxicology and environmental safety, 189, 109974.
Thatoi, H., Behera, B. C., Mishra, R. R., & Dutta, S. K. (2013). Biodiversity and
biotechnological potential of microorganisms from mangrove ecosystems: a review. Annals of Microbiology, 63(1), 1-19. Vethanayagam, R. R. (1991). Purple photosynthetic bacteria from a tropical mangrove environment. Marine Biology, 110, 161-163.
Vethanayagam, R. R., & Krishnamurthy, K. (1995). Studies on anoxygenic photosynthetic
bacterium Rhodopseudomonas sp. from the tropical mangrove environment.
Zhao, L., Zhao, C., Han, D., Yang, S., Chen, S., & Yu, C. P. (2011). Anaerobic utilization of
phenanthrene by Rhodopseudomonas palustris. Biotechnology letters, 33, 2135-2140.
ความคิดเห็น